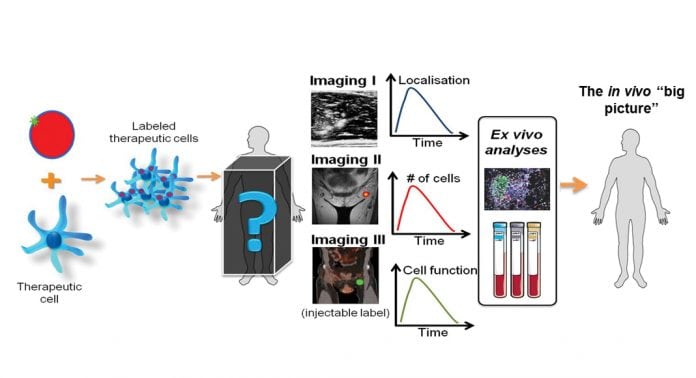
Dr Mangala Srinivas, from the Multiscale Imaging Lab, discusses some of the challenges facing cell therapies and how they might be overcome.
Cell therapy’ – the very name conjures the ability to regenerate ripped spinal cords, replace damaged tissue in the brain or in the heart, and eventually perhaps bring humanity closer to immortality. The near-miraculous public expectations of cell therapies have led to an underground stem cell tourism market, sometimes with disastrous consequences for these unregulated, dubious procedures.
Cell therapies consist of the transfusion of whole, live cells to a subject for treatment of a disease or condition. Thus, other than bone-marrow transplants, most cell therapies are experimental. The main types of cell therapies involve either immune cells, typically for the treatment of cancer, or stem cells in regenerative medicine. In the EU, cell therapies are classified as advanced therapeutic and medicinal products (ATMPs). Recent years have seen some clinical trials that have been spectacularly successful, and many more that have been ambivalent, and also some with negative results and even fatalities.
Why do cell therapies fail?
Cell therapies are extremely complex. In addition, each patient is very individual in their reaction to their treatment, in their disease stage and characteristics, in their treatment history, and in their ‘omics’ (such as their genome, proteome, microbiome), adding many further layers of complexity. A successful outcome is frequently defined by progression-free survival or factors such as improvement of functionality. In the short term, it may be possible to carry out blood tests for specific markers.
However, these measures do not assess the therapeutic cells directly. Instead, they are indirect measures of the cells. For example, transferred pancreatic islets in diabetic mice or patients may restore glucose homeostasis, at least for a time. But, is this due to most of the transferred islets surviving and functioning at a low level, or instead to very few islets surviving but functioning at a very high level? Such data is essential in order to truly optimise cell therapy for a patient and to understand the underlying biology.
A direct means of assessing transferred cells is essential
Success or failure measured through indirect means could be caused by several players, including many that are not related to the therapeutic cells themselves. The only direct means to monitor the transferred cells is often through an invasive biopsy. This is clearly not desirable, nor is it even always feasible.
Thus, how can cells be monitored directly?
Non-invasive, in vivo imaging techniques are an excellent solution, although with several caveats.
Non-invasive imaging could be a solution
Clinical non-invasive imaging modalities include ultrasound, computed tomography (CT, which uses X-rays), single photon emission tomography (SPECT, which needs a radioactive tracer), positron emission tomography (PET, which needs a radioactive tracer), and magnetic resonance imaging (MRI, which does not use ionising radiation, although contrast agents are often used). Fluorescence-based techniques are sometimes used, especially intra-operatively. In addition, photoacoustics or multispectral optoacoustic tomography (MSOT) is an emerging clinical modality. Preclinically, fluorescence imaging is often used in mice, as the penetration limits are less of an issue due to their small size.
Important factors to consider when selecting an imaging modality include:
- Location;
- Numbers;
- Migration;
- Kinetics;
- Timeframe (hours, days, weeks);
- Longitudinality (if needed);
- Resolution needed;
- Sensitivity desired; and
- Need for, or availability of, suitable imaging agent(s).
In addition, each imaging modality itself has factors that must be considered, such as:
- Cost;
- Availability;
- Imaging and set-up time;
- Patient considerations (general health, patient comfort);
- Availability of suitable imaging agents;
- Side effects of imaging agents, if any, on the patient;
- Exposure to radioactivity;
- Sensitivity;
- Resolution;
- Reproducibility;
- Operator dependence;
- Depth or tissue penetration limits, if relevant;
- Safety considerations;
- Quantifiability, if needed;
- Availability of cell labelling protocols;
- Availability of facilities and expertise for cell labelling for imaging, if needed;
- Any effect(s) of the imaging or imaging agent on the therapeutic cells;
- Factors relating to the imaging agent (such as cost, biodistribution, biological clearance, regulatory approvals);
- Non-specific or confounding signal(s); and
- Image interpretation.
For example, CT is often the workhorse of clinical imaging, but it is not used for cell imaging. Thus far, mainly PET, SPECT and MRI have been used for cell tracking in the clinic, and the work with MRI remains experimental. Preclinically, many studies rely on genetically modified cells expressing fluorescent proteins or other reporter genes for imaging. However, genetic modification for reporter gene expression is not easily translated to the clinic.
It is important to keep in mind that specific imaging of therapeutic cells is a relatively new field, and it has come to the fore due to the great interest in cell therapies and the need to optimise and personalise the treatments. There are no established protocols for therapeutic cells and a dearth of commercial imaging agents designed specifically for cell tracking.
Moreover, every imaging modality has specific strengths and weaknesses. For example, PET may be the most sensitive, but its need for radioactive tracers can limit its applicability. MRI does not need radiolabels and could be used to track cells for longer periods of time, but it lacks sensitivity and it can be difficult to ascertain that the label remains with the relevant cells, especially at later time points. To overcome these issues, a combination of imaging modalities is often used, i.e. multimodal imaging. Preclinically, multimodality is often a combination with a fluorescent dye, as fluorescence allows for histological confirmation of the imaging data, in addition to cheap and easy in vivo fluorescence imaging or tomography. Clinically, cell tracking has only been carried out with single modality agents in the handful of trials that have been completed so far.
Regulatory clearance
Obtaining regulatory clearance for a new imaging label is an endeavour. Furthermore, there can be ambiguity on how to classify imaging agents, as well as imaging agents within therapeutic cells. Standard protocols used in drug trials simply do not apply here. On a positive note, regulatory bodies are aware of such issues and are typically willing to work with researchers to identify pathways together.
Costs and patient burden
Cell therapies can be very costly. Adding imaging sessions, imaging agents, and the time and expertise for cell labelling will further increase the immediate cost. Patient burden also goes up, due to the need for imaging sessions. Although these factors tend to be relatively minor, they do need to be considered. Scheduling, in particular, between the imaging staff and the cell therapy administrators can be challenging.
Interdisciplinary co-operation
Finally, the imaging of cell therapies is a vast and highly interdisciplinary undertaking. It needs expertise in imaging, imaging hardware, image analysis, patient management, cell therapies, imaging agent development, cell biology, pharmacists, chemists, and clinicians.
The big picture
With all this in mind, my group at the Multiscale Imaging Lab is working on the development of a multimodal imaging platform for cell tracking. This platform consists of nanoparticles that can be customised for different cell types. The native nanoparticles are visible in MRI, MSOT and ultrasound, and can be modified for fluorescence, SPECT and PET. They can be made fully GMP-grade for clinical use. The use of a modifiable platform may simplify the regulatory pathways due to the overlap between different versions of the particles. For preclinical use, further modifications such as the inclusion of specific drugs or active agents, or the modification of the particle surface, diameter or charge, are possible. This has led to the setting up of a spin-off as well.
Fig. 1 summarises the benefits that imaging, particularly multimodal imaging, can offer towards the optimisation of cell therapies. Getting the ‘big picture’ may require a combination of imaging modalities, and the use of conventional techniques such as histology and blood tests, to validate the in vivo data. However, the ability to directly monitor and perhaps adapt the treatment as needed for each individual patient could make the crucial difference between success and failure.
Dr Mangala Srinivas
Assistant Professor
Department of Tumor Immunology
Radboud Institute for Molecular
Life Sciences
Radboud University Medical Center
www.multiscaleimaging.com
This is a commercial article that will appear in Health Europa Quarterly issue 6, which will be published in August, 2018.