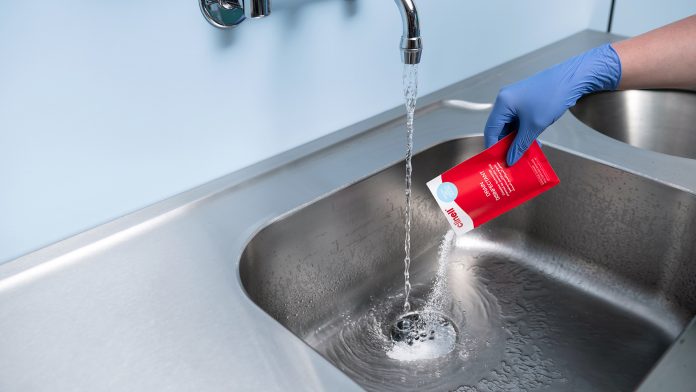
In response to increasing links between outbreaks and contaminated drains, GAMA Healthcare explores emerging evidence which supports the use of peracetic acid in the management of drain biofilms.
In 1972, researchers documented the link between contaminated sinks and pathogen transfer when other transmission methods could not explain the source of Pseudomonas aeruginosa infections and their fatal effect in a Burns Hospital.1
The usual suspects (contaminated hands and patient-to-patient spread) had already been ruled out; it was then that clinical researchers began to look beyond the surface… and down the drain.
Back in the 1970s, with the protective arm of antibiotics and the emerging power of infection prevention, managing infections looked promising. However, as we find ourselves in 2023, antimicrobial resistance is an epidemic, and we cannot lean on treatment alone; prevention is key.
The surfaces we cannot see
There are no avoiding drains in healthcare settings. Sinks are crucial to delivering care and infection prevention practices like hand hygiene. However, these interventions nurture sinks with everything they need to grow biofilms.
Hand washing, a measure known to reduce the burden of infection transmission between patients, healthcare workers and the environment, is feeding drainage systems with microorganisms that can adapt to live in these hidden environments.
Down the drain and out of sight, microorganisms are more challenging to remove. Unfortunately, unlike typical surfaces, they cannot be simply wiped away. Furthermore, hidden in drains’ optimum conditions, microorganisms can leverage another survival strategy – forming biofilms.
How do bacteria stick?
Until the late 1970s, bacteria were thought of as lonely single-celled microorganisms – a perception that perhaps still exists within groups less familiar with the complex, microscopic world that lives through the lens of a microscope.
The world’s understanding of bacteria changed with the publication of How Bacteria Stick in 1978, the first publication to describe ‘biofilms’.2
Now, we know that up to 80% of all living cells on our planet exist in biofilms.3
Instinct to survive
Like most living organisms, bacteria are driven by survival and the need to find food, water, and shelter. Forming biofilms is one tactic bacteria can deploy to answer all these needs.
Described as complex communities of microorganisms immersed in extracellular polymeric substances (EPS), biofilms develop in a series of reversible steps:
- Planktonic: free-floating bacteria approach a surface
- Sessile: individual bacterium attach to the surface
- Biofilm: attached bacteria secrete EPS as the biofilm grows
- Dissemination: environmental and physiological cues can cause bacteria to exit the biofilm and re-enter the environment
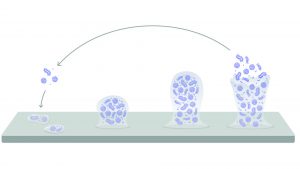
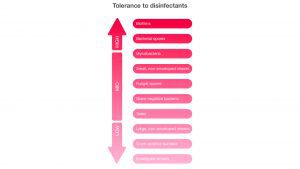
Top of the tolerance table
As single cells, planktonic bacteria have limited options to help them survive the harsh environment. For healthcare professionals, this has worked to our advantage in eliminating microbial contamination and infection from our hospitals.
Fortunately for them, these planktonic bacteria can leverage age-old survival mechanisms by changing state to form spores or biofilm, which are much more challenging to eradicate.
Unfortunately, bacteria sheltered within a biofilm are significantly more difficult to kill with chemical disinfectants (Fig. 2). Biofilms’ tolerance to disinfectants and their impact on healthcare tell us that the correct treatment must be used to manage the threat of biofilms.
In healthcare, chlorine has been widely adopted as the go-to treatment for drain biofilms. While it can kill free-floating bacteria, chlorine underperforms in drain biofilm treatment compared to peracetic acid.5
Surviving disinfectant
As well as protection from the biofilm’s structure, microorganisms within can leverage another physical advantage by spontaneously altering their metabolism.
Due to the complex 3D form of a mature biofilm, fundamental necessities like oxygen, nutrients and other metabolites are less abundant to bacteria near the surface. The lack of these essentials gives rise to persister cells.
These persister cells, named so because they can ‘persist’ or survive antimicrobial agents, are dormant compared to their peers. In a 2020 review, persister cells were described as a ‘minor subpopulation of bacteria that are transiently tolerant to the lethal activity of antimicrobials’.6
The formation of persister cells may be another reason biofilms can recover after disinfection.7 That is why it is crucial to ensure that biofilms are treated with the most effective chemical agent.
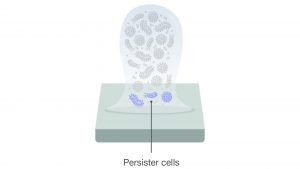
Detecting drain biofilms
Once biofilms have been established in a drainage system, removing them is almost impossible. Not only do biofilms, and their inhabitants, have highly polished survival mechanisms, but they are also difficult to detect – until it is too late.
Unfortunately, biofilms are challenging to detect unless samples of the surface themselves are cultured and analysed. However, sometimes biofilms are located in response to outbreaks with no apparent source.
Tracing the source of outbreaks
In a review that analysed 23 outbreaks of carbapenem-resistant microorganisms, wastewater and drainage systems were concluded to play an essential role in the transmission of infections in the healthcare environment.8
Contaminated drains are a known source of infection, posing a threat, particularly to vulnerable patients receiving care. In fact, a 2021 study of 73 intensive care units (ICU) showed that over half of all sinks are contaminated with a multi-drug-resistant microorganism.9
What is being done to prevent outbreaks?
With increasing links between outbreaks and contaminated drains, hospitals have made significant changes to their infrastructure to mitigate the risk, with some going to the extent of tearing out pipes and sinks in place of new ones. However, replacing hardware is costly if hospitals want to stay on top of the biofilm threat.
We now know how biofilms seed microorganisms back into the environment due to physical disruption, such as running water which causes splashback. This has driven some hospitals to introduce further changes to the clinical environment to stop transmission by separating sinks/drains from patients and healthcare workers.
However, with successful chemical disinfection, the threat of drain biofilms can be eradicated and managed long-term – without the need for costly physical interventions.
Selecting a chemical disinfectant
Researchers at Cardiff University, UK, analysed the effect of multiple disinfectant candidates for biofilm management.5
Biofilms’ ability to recover after disinfection is one factor that should influence how they are treated and which chemical agents they are treated with. That is why both log reduction and regrowth were considered.
- Log reduction gives the number of bacteria that are killed or removed after treatment; and
- Regrowth is the time needed by the biofilm to recover after treatment.
Peracetic acid outperforms chlorine
Two models, which represented common drainage systems (U-bend and trap), were constructed to help researchers analyse the effect of different disinfectant protocols.
Each model was divided into three sections (front, middle, and back) to monitor the effect throughout the system.
The resulting data (Fig. 4) showed peracetic acid, the active ingredient in Clinell Drain Disinfectant, outperformed traditional chlorine-based protocol in all three sections.
Most notably, peracetic acid prevented biofilm regrowth throughout the drainage system, proving that a simple, regular protocol could effectively manage biofilm without tearing out hardware.
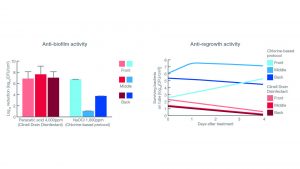
What next?
Evidence demonstrates a strong case for the use of peracetic acid in the management of biofilms in healthcare.
In ICUs alone, six in ten sinks are inadequately disinfected or not disinfected at all.9 Therefore, the need for a simple-to-use, the effective protocol is critical to minimising the risk of biofilms in the future.
For more information about Clinell Drain Disinfectant, visit www.gamahealthcare.com/clinell-peracetic-acid-range
References
- Edmonds P, Suskind RR, Macmillan BG, Holder IA. Epidemiology of Pseudomonas aeruginosa in a Burns Hospital: Evaluation of Serological, Bacteriophage, and Pyocin Typing Methods. Appl Microbiol. 1972;24(2):213-218. doi:10.1128/am.24.2.213-218.1972
- Costerton JW, Geesey GG, Cheng KJ. How Bacteria Stick. Sci Am. 1978;238(1):86-95. doi:10.1038/scientificamerican0178-86
- Flemming HC, Wuertz S. Bacteria and archaea on Earth and their abundance in biofilms. Nat Rev Microbiol. 2019;17(4):247-260. doi:10.1038/s41579-019-0158-9
- McDonnell G, Burke P. Disinfection: is it time to reconsider Spaulding? J Hosp Infect. 2011;78(3):163-170. doi:10.1016/j.jhin.2011.05.002
- Ledwoch K, Robertson A, Lauran J, Norville P, Maillard JY. It’s a trap! The development of a versatile drain biofilm model and its susceptibility to disinfection. J Hosp Infect. 2020;106(4):757-764. doi:10.1016/j.jhin.2020.08.010
- Kaldalu N, Hauryliuk V, Turnbull KJ, la Mensa A, Putrinš M, Tenson T. In Vitro Studies of Persister Cells. Microbiol. Mol. Biol. Rev. 2020;84(4). doi:10.1128/MMBR.00070-20
- Jiang T, Li M. Quorum sensing inhibitors: a patent review. Expert Opin Ther Pat. 2013;23(7):867-894. doi:10.1517/13543776.2013.779674
- Carling PC. Wastewater drains: epidemiology and interventions in 23 carbapenem-resistant organism outbreaks. Infect Control Hosp Epidemiol. 2018;39(8):972-979. doi:10.1017/ice.2018.138
- Valentin AS, Santos S dos, Goube F, et al. A prospective multicentre surveillance study to investigate the risk associated with contaminated sinks in the intensive care unit. Clin Microbiol Infect. 2021;27(9):1347.e9-1347.e14. doi:10.1016/j.cmi.2021.02.018
This article is from issue 25 of Health Europa Quarterly. Click here to get your free subscription today
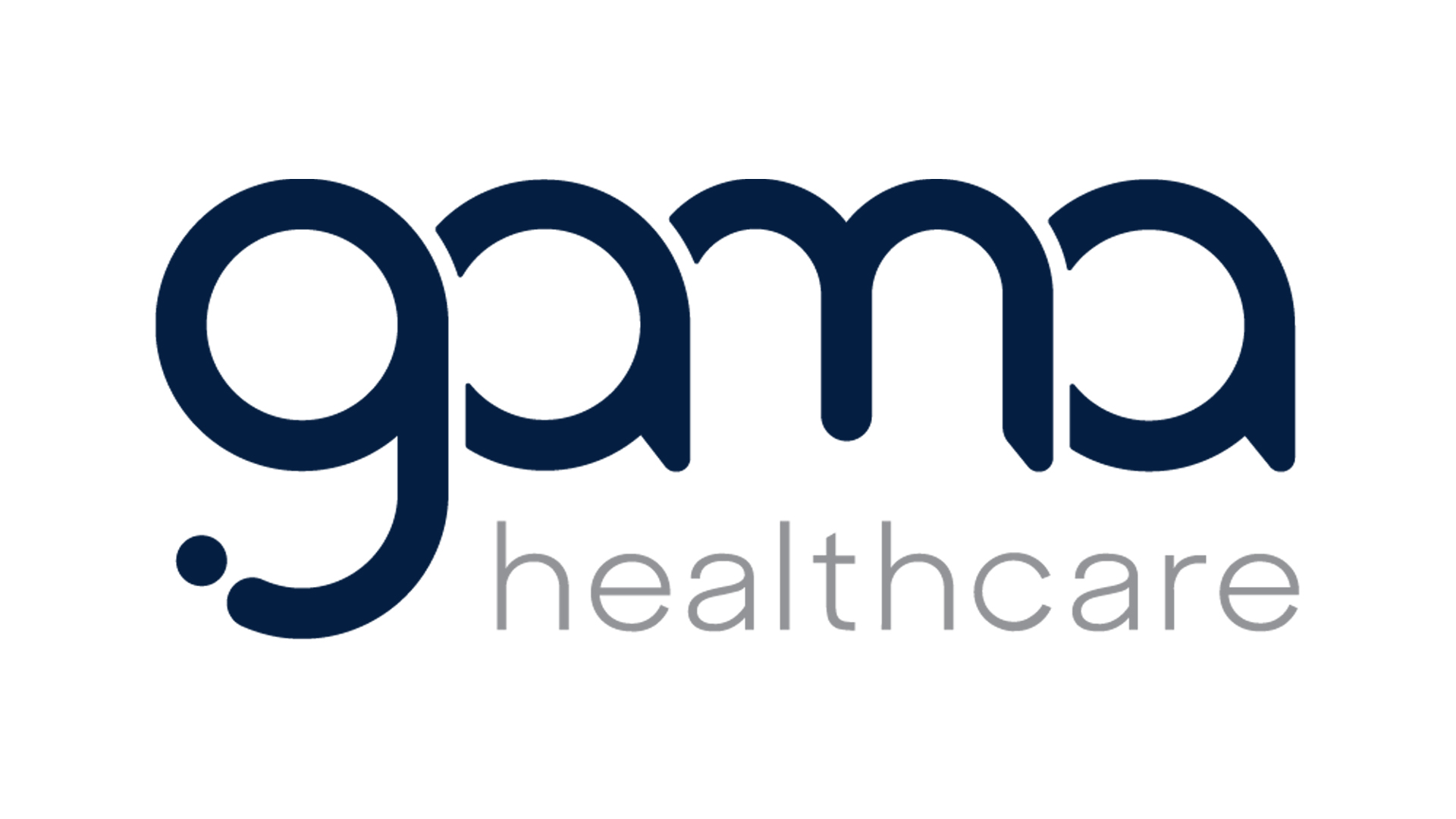