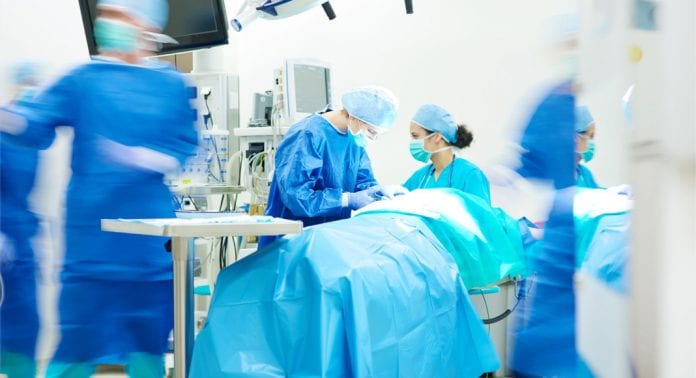
Exploring the risks of reopening operating rooms under the shadow of COVID-19 with Sue Barnes, RN, CIC, FAPIC, Infection Prevention Consultant.
The SARS-CoV-2 virus, which has caused the current COVID-19 pandemic, is a new airborne risk in operating rooms (ORs).1 During the first months of the pandemic, elective surgical procedures were cancelled in many ORs, but more recently have begun to reopen. This reopening process is guided by a multidisciplinary joint position statement recommending measures to prevent ongoing transmission of SARS-CoV-2.2
Some of these measures will serve not only to prevent transmission of SARS-CoV-2 and other respiratory viruses, but also will reduce the risk of contaminated particulates which can cause surgical site infections. For example, the joint statement recommends fewer surgical team members in the OR during cases, which reduces the number of individuals shedding bacteria laden skin cells and contaminated hair fibres. Other measures focusing only on prevention of COVID-19 include the use of N95 respirators plus face shield or powered air-purifying respirators (PAPR) during intubation and extubation of COVID patients. Prevention of transmission is of paramount importance, especially considering the ongoing restricted supplies of personal protective equipment (PPE).
OR air quality and surgical infection risk in 2020
Professional organisations provide guidance regarding air quality in United States (US) ORs in order to reduce the risk of surgical site infections, including Association for Operating Room Nurses and Associates (AORN), Centers for Disease Control and Prevention (CDC), American National Standards Institute (ANSI), American Society for Healthcare Engineering (ASHE), and American Society of Heating, Refrigerating and Air Conditioning Engineers (ASHRAE). These organisations recommend engineering controls that regulate dilution of air (20 air changes per hour), filtration of air (Minimum Efficiency Reporting Value [MERV] filtration rating between 13 and 14), and pressurisation (positive to surrounding areas).3,4
Since the organisations are not regulatory, they cannot mandate these engineering controls, but their guidance is still voluntarily followed by the majority of ORs. In addition, AORN and CDC recommend certain processes that can further help to reduce airborne contaminants and the associated surgical infection risk, including minimising foot traffic during cases, long sleeves and full head hair coverage for surgical team members. They also recommend minimising door openings in the OR during cases but provide no parameter regarding how many, if any, door openings are acceptable. More recently, AORN has added a recommendation for patient hair removal from the surgical site prior to when the patient enters the OR in order to prevent the dispersal of bacteria-laden clipped hair in the sterile OR environment.5
These prevention measures are necessary because of the massive numbers of skin scales that are continually being shed from the body surface. Many of these skin scales carry microorganisms including Staphylococcus aureus and methicillin-resistant Staphylococcus aureus (MRSA) which, when they are present in an OR, can cause surgical site infections (SSI).6 As many as 10 million of these skin particles can be shed in a 24-hour period, detached by movement as well as friction between skin and clothing.7
Surgical procedures involving an implant have the greatest risk of infection from airborne contaminants.8 Implant procedures of many types are becoming ever more common, including hip and knee replacements, hernia repair with mesh, breast implants and many more. Almost every surgical speciality now performs some type of procedure involving implanting a medical device. Because these implants are foreign bodies, very few organisms are necessary to trigger the growth of biofilm which can lead to an infection.9
Bacteria circulating in OR air can come from a number of sources. They can be attached to skin cells, textile and hair fibres, as well as respiratory aerosols shed by members of the surgical team. These can be dispersed in the OR on air currents; and if they settle in the open incision or adhere to the implant, they can cause post-operative SSI.10 Many studies have demonstrated the relationship between airborne bacteria and SSI risk.11-13 As airborne bacterial levels increase in ORs, the risk of infection also increases. This is further impacted by the number of people in the room. Airborne contamination has been reported to account for between 20% and 24% of SSIs.13
However, despite these prevention measures and controls, zero preventable SSI remains an elusive goal for most ORs. SSIs continue to occur in 2% to 4% of all patients undergoing surgical procedures, remaining a significant cause of morbidity and mortality after surgery.14 In addition, these infections are a major economic burden, with a joint replacement infection estimated to result in costs of more than $400,000 per case.15 The costs are due to prolonged hospitalisation, investigation resources, treatment and re-operation – but more importantly, the infections affect the quality of life of the patient and their family.
Surprisingly, given the risks and costs associated, there are no requirements for measuring and maintaining air quality in US ORs, though there are in US compounding pharmacies. Instead, the engineering controls described above are considered sufficient to ensure safe air quality for patients and surgical teams.4 However, these engineering controls (positive air pressure, increased air changes, temperature and humidity control and high efficiency particulate air filter [HEPA]) can be defeated by door openings and room traffic during cases.16 As in US compounding pharmacies, air quality standards have been established for European ORs17, where there are bacteria and particle limits and a standard methodology for testing air quality. In the US, compounding pharmacies comply with International Standards Organization (ISO) Class 5 standards for air quality, which permit a maximum of 105 particles per cubic metre of air, which must be measured and tracked.18
Surgical smoke plume and employee health risk
Operating rooms create airborne risk for surgical team members as well as patients as the result of exposure to smoke plume, which has been reported to cause upper respiratory irritation, human papilloma virus (HPV) infection, and squamous cell carcinoma.19 Within the US, an estimated 500,000 healthcare workers annually provide or assist with procedures that generate laser or electrosurgical smoke.20 Surgical smoke plume is generated when a surgeon uses an electrosurgical unit or a laser for thermal destruction of tissue. This smoke can contain carbon monoxide, viral fragments, bacterial fragments, carcinogenic and mutagenic particles, which all create health risks for both the surgical team members and the patient.21,22
According to one study, exposure to surgical smoke generated by cautery/burning of one gram of tissue is comparable to the mutagenic effect of smoking three cigarettes for lasers, and six cigarettes for electrocautery.23 This study and others have concluded that surgical masks do not provide adequate protection from smoke plume. Smoke evacuation devices are designed to be located within two inches of smoke generation in order to provide effective protection of surgical team members and patients.24 Unfortunately, smoke evacuation devices are not consistently used in all ORs.25
Technology designed to improve the quality of OR air
SSIs resulting from intra-operative contamination are primarily due to airborne particles carrying microorganisms, mainly Staphylococcus aureus, which can settle on the open wound, the surgeon’s hands and instruments.26 Given this, and the elusive goal of zero preventable surgical site infections, looking beyond engineering controls in the OR would be prudent.
Adjunctive technologies such as advanced air filtering technology and UV disinfection have been employed to supplement engineering controls in the OR. However, the combination of HEPA and UV-C disinfection of air has been demonstrated to be especially effective in reducing airborne particles, circulating pathogens, and reducing surgical infection rates.15, 27-32
One such proven device is the Illuvia by Aerobiotix. This is a wheeled, enclosed free-standing air decontamination unit with a small footprint. It provides both HEPA filtration and ultraviolet germicidal irradiation to the airstream without disruption of the air flow pattern in ORs. The unique design of the Illuvia includes a built-in particle counter, which can be used to validate the efficacy of air cleaning before, during and after surgical cases. As mentioned, European ORs adhere to a maximum particle limit for the OR; and with Illuvia’s built in particle counter, this could be used for measurements during cases, not just before or after.18
The Illuvia has undergone independent laboratory testing, demonstrating 100% inactivation efficiency in viral single-pass testing, using the surrogate MS2 virus at 450 cubic feet of air per minute. This testing was reviewed by the FDA in 2018. Coronavirus is larger than MS2, making it potentially more vulnerable to ultraviolet and mechanical filtration than the MS2 test organism. In hospital studies, after the Illuvia was employed in two different orthopaedic ORs, it reduced the circulating airborne bacterial level by 80%; while in a general surgery OR the airborne bacterial level was reduced by 67%.28,31
In a March 2018 cross-sectional study at Siena University Hospital (Italy), the Illuvia was positioned with the intake close to the main door (sideways) and the output directed towards the centre of the OR. The experiment was designed to test the impact of the device on air quality. Particle counts were measured during three consecutive periods during a bariatric surgical case where the OR door was opened eight, nine and four times, respectively (the device was on for the second period only).
The device demonstrated efficacy in reducing airborne particles, including those ≥0.5 µm which is commonly used as a reference for environmental contamination. There was a statistically significant reduction (p < 0.05) in the number of particles from ≥0.3 µm to ≥10 µm particles when the device was on. This outcome was achieved despite the presence of 10 OR staff and the door openings as described. The most common environmental measures currently employed in ORs for ensuring OR air quality, UV systems and engineering controls, do not ensure a reduction in the number of airborne particles or bacteria in the room, although they do increase costs.13 Given all these aspects, the investigators concluded that the Illuvia could help reduce the probability of SSI by reducing airborne particulates that may be vectors of pathogenic bacteria.32
Successful introduction of new technology such as the Illuvia in ORs often involves a surgeon champion who can shepherd the business case through all required committees and approval processes. To further support successful introduction, the Aerobiotix team provides a flexible template acquisition model which eliminates the need for capital expenditure. Avoiding capital level expense automatically reduces much of the required approvals.
The primary differences between Illuvia and other air filtration or UV technologies on the market is that the Illuvia is small and mobile, does not disrupt the air flow pattern, requires no installation and also includes two filter banks. It not only kills circulating microorganisms using UV-C, but also filters out the particles, some of which can be hazardous, such as byproducts of surgical smoke.
Infection prevention programmes are dynamic; and the most successful include early adopters of new technology with proven efficacy. Looking beyond standard engineering controls is critical given the increasing airborne risks to patients and surgical teams, including the newest, COVID-19. As we continue the journey towards zero preventable surgical infections, ongoing study in this area of risk will help to determine the best method(s) for optimising air quality in ORs. To learn more about Illuvia, visit www.aerobiotix.com.
References
1 Wilson NM, Norton A, Young FP, Collins DW. Airborne transmission of severe acute respiratory syndrome coronavirus-2 to healthcare workers: a narrative review [published online ahead of print, 2020 Apr 20]. Anaesthesia. 2020;10.1111/anae.15093. doi:10.1111/anae.15093
2 Joint Statement: Roadmap for Resuming Elective Surgery after COVID-19 Pandemic; Online April 17, 2020. American College of Surgeons American Society of Anesthesiologists Association of periOperative Registered Nurses American Hospital Association. https://www.facs.org/covid-19/clinical-guidance/roadmap
-elective-surgery. Accessed July 8, 2020.
3 JCAHO Hospital Accreditation Standards; 2004 Environment of Care 7.10 -No. 15.
4 ASHRAE 170: https://www.techstreet.com/ashrae/standards/ashrae-170-2017?gateway_code=ashrae&product_id=1999079. Accessed July 8, 2020.
5 Edmiston CE Jr, Leaper DJ, Barnes S, et al. Revisiting Perioperative Hair Removal Practices. AORN J. 2019;109(5):583-596. doi:10.1002/aorn.12662
6 Clark RP, de Calcina-Goff ML. Some aspects of the airborne transmission of infection. J R Soc Interface. 2009;6 Suppl 6(Suppl 6):S767-S782. doi:10.1098/rsif.2009.0236.
7 Lidwell OM, Lowbury EJ, Whyte W, Blowers R, Stanley SJ, Lowe D. Airborne contamination of wounds in joint replacement operations: the relationship to sepsis rates. J Hosp Infect. 1983;4(2):111-131. doi:10.1016/0195-6701(83)90041-5
8 Petrova, O.E. and K. Sauer, Sticky situations: key components that control bacterial surface attachment. J Bacteriol, 2012. 194(10): p. 2413-25.
9 Zimmerli W, Trampuz A, Ochsner P. Prosthetic-Joint Infections. N Engl J Med. October 14, 2004; 351:1645-1654.
10 Owers KL, James E, Bannister GC. Source of bacterial shedding in laminar flow theatres. J Hosp Infect. 2004 Nov;58(3):230-2.
11 Eickhoff, T. C. (1994). Airborne nosocomial infection: a contemporary perspective. Infection Control and Hospital Epidemiology. 15(10): 663-672.
12 Durmaz G et al. The relationship between airborne colonisation and nosocomial infections in intensive care units. J Chemotherapy. 39: 465-471
13 Kowalski W. Ultraviolet Germicidal Irradiation Handbook. Springer Verlag, Berlin 2009. pp 399-418.
14 Agency for Healthcare Research and Quality (AHRQ) Patient Safety Network online. Surgical Site Infections. September 2019. https://psnet.ahrq.gov/search?f%5B0%5D=safety_target%3A4830 Accessed July 8, 2020.
15 Parvizi J et al. Environment of care: is it time to reassess microbial contamination of the operating room air as a risk factor for surgical site infection in total joint arthroplasty? American Journal of Infection Control. 2017 Nov 1;45(11):1267-1272.
16 Perez P, Holloway J, Ehrenfeld L, et al. Door openings in the operating room are associated with increased environmental contamination. Am J Infect Control. 2018;46(8):954‐956.
17 Charkowska, A. “Ensuring Cleanliness in Operating Theatres”. Jose 2008, Vol. 14, No. 4 pp. 447–453.
18 Clean room classifications and ISO standards https://www.americancleanrooms.com/cleanroom-classifications/ Accessed July 8, 2020.
19 Fletcher JN, Mew D, Descôteaux JG. Dissemination of melanoma cells within electrocautery plume. Am J Surg. 1999; 178(1):57-59.
20 OSHA Laser/Electrosurgery Plume (n.d.) https://www.osha.gov/SLTC/laserelectrosurgeryplume/index.html Accessed July 8, 2020.
21 Schultz L. An analysis of surgical smoke plume components, capture, and evacuation. AORN J. 2014;99(2):289-298.
22 Bree K, Barnhill S, Rundell W. The dangers of electrosurgical smoke to operating room personnel: a review. Workplace Health Saf. 2017;65(11):517-526.
23 Calero L, Brusis T. Laryngeal papillomatosis — first recognition in Germany as an occupational disease in an operating room nurse. Laryngorhinootologie. 2003;82(11):790-793.
24 Goon PKC et al. Virus induced cancers of the skin and mucosa: are we dealing with smoking guns or smoke and mirrors in the operating theatre? Dermatol Ther. 2017 Jun;7(2):249-254.
25 Schultz L. Can efficient smoke evacuation limit aerosolisation of bacteria? AORN J. 2015;102(1):7-14.
26 Chauveaux D. Preventing surgical-site infections: measures other than antibiotics. Orthop Traumatol Surg Res. 2015;101(1 Suppl):S77-S83. doi:10.1016/j.otsr.2014.07.028
27 Bischoff W et al. Impact of a novel mobile air purification system on the bacterial air burden during routine care. Oral presentation SHEA Conference. Spring 2018.
28 Curtis G et al. Reduction of particles in operating room using UV air disinfection and recirculation units. The Journal of Arthroplasty. (2017) 1-5.
29 Davies GS, Bradford N, Oliver R, Walsh WR. The effects of a novel decontamination-recirculating system in reducing airborne particulate: A laboratory-based study. Oral presentation: The European Bone & Joint Infection Society Conference. Nantes France Sept 7-9, 2017.
30 Gannon C et al. Reduction of total and viable air particles in the OR setting by using ultraviolet in-room air disinfection and recirculation units. American Association of Hip and Knee Surgeons Conference. November 4, 2017.
31 Kirschman D, Eachempati S. Airborne bacteria in the operating room can be reduced by HEPA/Ultraviolet air recirculation system (HUAIRS). Presented at the Surgical Infection Society (SIS) – 37th Annual Meeting. May 2 – 5 2017 in St. Louis, MO.
32 Messina G et al. A mobile device to reduce airborne particulate and prevent surgical site infections. European Health Association Conference. Marseille France November 20-23, 2019.
Sue Barnes, RN, CIC, FAPIC
Independent IP Consultant
www.zeroinfections.org
This article is from issue 14 of Health Europa. Click here to get your free subscription today.