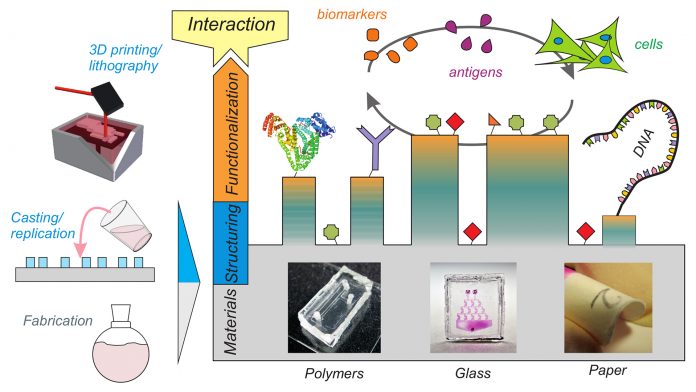
Dr Bastian Rapp of Karlsruhe Institute of Technology’s NeptunLab explains how material research is key to the future of healthcare research
Healthcare research is situated at the interface between chemistry, biology and engineering sciences. In terms of chemical composition, even the tiniest organisms or biological environments are highly complex. In healthcare research, biomolecules and organisms are taken out of their natural environments and studied/reacted/manipulated in an artificial environment that has to be as complex as necessary to facilitate correct biochemical, chemical and biological function while still being technically as simple as possible to allow control and readout. Surfaces play a major role in the interactions between biomolecules or organisms and artificial substrates. Surfaces are characterised by the choice of substrate material, their overall geometry or structure (cavities, channels), as well as their customised surface biofunctionalisation (i.e. attachment of biomolecules).
Every molecular interaction study, every cell-experiment, every detection device needs a specific experimental set-up and therefore a special chip design, structuring and surface functionalisation. Miniaturisation is a major factor for healthcare research to ensure minimum reagent costs and waste generation, high-throughput and statistically significant results, as well as portable analysis tools such as paper analysis strips. Structuring techniques such as lithography or direct machining, as well as the 21st Century revolution in 3D printing, provide new opportunities for ‘well-known materials’, i.e. materials that have been successfully used for many years in healthcare research in cell-culture, chip fabrication or for biofunctionalisation, for example.
Using well-known materials ensures that questions such as long-term stability and biocompatibility do not restrict usage of the material. The choice of material for the fabrication of analytical devices such as chip, test strips or point of care devices should always be motivated by the application at hand, its restrictions and prerequisites. In reality, however, the choice of material is often motivated by the ease and cost of manufacturing and the associated machining, and personal overhead costs. There is often a large discrepancy between the needs and requirements of research and industry. Research usually requires customised designs, material processing and structuring, which allow fast experimental iteration and optimisation. Industry usually requires manufacturability via scalable methods such as polymer replication.
Our laboratory is dedicated to providing novel material and manufacturing platforms, i.e. fabrication, structuring and functionalisation tools that enable the generation of specialised chips and microenvironments in a fast and effective manner from a broad spectrum of materials. Our vision is that customised, carefully functionalised lab-on-chip devices will be fabricated on-demand by the researchers or by a closely associated manufacturer from any desired material in the near future fast and reliably.
NeptunLab’s techniques
Our material platforms tools are divided into novel material fabrication, material structuring, and material functionalisation techniques (Fig. 1). Here we present six material platforms with polymers, glass and paper that each improves the state-of-the-art with an emphasis on simplicity and applicability. We have developed novel fabrication techniques for polymers like polystyrene (PS) and polymethylmethacrylate (PMMA) that enable the direct structuring of these materials by standard replication methods.
Polymers, however, are not the only material of interest. For numerous applications where optical transparency and chemical resilience are of supreme importance, glass is the material of choice. We have invented a novel technique for glass structuring, allowing for 3D printing of transparent fused silica glass parts on standard stereolithography machines. In terms of material structuring we developed a novel technique for polydimethylsiloxane (PDMS) structuring, that allows for printing of 3D channel structures directly into the bulk material, for e.g. microfluidic applications. Our research further focuses on disposable paper-based analytical devices where we are looking into new ways of paper structuring by hydrophilic/hydrophobic functionalisation. In terms of chemistry, the surfaces of all these materials must be fine-tuned to match the properties of the desired healthcare application. We have described a reliable and fast functionalisation technique that allows for the attachment of any desired biomolecule (protein, DNA, antibodies) on any of the above mentioned materials. This technique completes our platform portfolio and allows us to produce customised healthcare tools for applications such as cellular assays, microfluidics and bioanalytics such as paper test strips.
Polystyrene and PMMA processing
Polystyrene and PMMA are two of the most common polymers used in biochemical and healthcare research. Most cell-culture and biochemical equipment is made from PS, which is known for its biocompatibility and long-term stability. PMMA is one of the least hydrophobic polymers, which makes it especially interesting for highly integrated microfluidic devices that are difficult to fill via capillary forces if a more hydrophobic polymer is used. PMMA is also an excellent choice for optical applications due to its optical properties. PS and PMMA processing and structuring usually relies on industrial-scale processes such as hot embossing and injection molding.
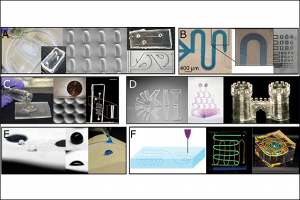
In order to make PS available for bench-top fabrication processes, and thus accessible for research use, we have developed a new form of PS which we term ‘Liquid PS’. This innovation can be structured in a simple ‘pour-and-cure’ casting technique. Liquid PS is a viscous pre-polymer mix that can be polymerised by light, resulting in pure PS (Fig. 2A). It possesses the same optical, mechanical and chemical properties as commercially available polystyrene and matches cell culture grade PS in terms of biocompatibility (Fig. 4A). This approach to a well-known material is attractive whenever the fabrication of molds or master structures for polymer replication is not viable, e.g. in the design phase. We have provided a likewise approach resulting in ‘Liquid PMMA’, which is again a liquid formulation that can be structured via direct lithography by light. The material allows the generation of high-resolution components in PMMA such as microfluidic mixers, complex channel networks or optical components, for example (Fig. 2B).
A revolution in glass processing
Glass is a highly versatile material due to its optical clarity and chemical resilience. However, glass and high-purity fused silica glass are notoriously difficult to structure. The most commonly used high-resolution structuring techniques are etching processes based on hydrofluoric acid, which requires special laboratories and equipment. Also, the freedom of design is limited due to the inherent isotropy of the etching process. We have recently developed two silica nanocomposites that contain large amounts of amorphous silica nanoparticles suspended in a small amount of organic binder matrix. One of the composites is a casting formulation which can be poured over a replication mold and hardened by light or heat exposure (Fig. 2C). The second composite can be structured in conventional 3D stereolithography printers to result in any desired structure (Fig. 2D). Once the part is produced by either of these methods, the component is heated to remove the organic binder matrix and to sinter the silica nanoparticles to a full-density transparent fused silica glass. The resulting material is identical to commercially fused silica glass in terms of physical, chemical and mechanical properties such as density, optical transparency, thermal resistance and wetting properties. Smoothness values of Ra ~2-5nm can be obtained in this process.
The printing of micro-scale parts as well as macroscopic centimetre-scaled objects is also possible. Using greyscale lithography, extremely smooth curved surface features such as microlenses can be produced. Glass has many potential applications in health, including components for analytics, diagnostics and sample treatment, cell-culture devices and optical components in endoscopes or surgical instrumentation.
Structuring polydimethylsiloxane
Polydimethylsiloxane (PDMS), a clear, elastomeric polymer, is the material of choice for most rapid prototyping approaches in microfluidics. This is due to it being cheap and easy to handle. It is usually shipped as a two-component formulation that cures after mixing at room temperature. It replicates small features with high fidelity and, due to its elasticity is convenient to handle during demoulding. However, 3D channel structures in PDMS can only be produced in a layer-by-layer fashion or by immersing a soluble 3D structure in a PDMS bulk with consecutive dissolving of the structure. Also, most fabricated channels possess square-shaped cross-sections due to the classical fabrication approach by moulding and consecutive sealing. However, circular channel cross-sections are significantly more relevant to healthcare research because they mimic the structure of blood vessels in vivo.
We have developed a method to print three-dimensional channels directly into uncured PDMS (see Fig. 2F), which we term ‘Suspended Liquid Subtractive Lithography’ (SLSL). For this, a fused deposition modelling (FDM) 3D printer is equipped with a syringe and a capillary needle through which a surfactant is pumped into the matrix. Due to the insolubility of the surfactant in the PDMS and the close match in surface energies, the two liquids do not mix, and instead of forming singular droplets, they create continuous surfactant ‘threads’ inside the PDMS body. After the PDMS is cured, the surfactant is removed by pumping and washing. This technique leaves a very smooth, completely circular channel inside the PDMS matrix – the first truly three-dimensional structures with circular and smooth channel walls created directly inside PDMS. This technology opens up various applications in biomimetics such as vascular networks to study cell behaviour in an in vivo-like environment.
Paper-based analytical devices
Bioanalytics and point-of-care diagnostics are growing markets in healthcare and medicine. Besides applications in the industrial world, these technologies become ever-more important for low-resource settings where water, electricity and reagents are scarce. Paper-based analytic devices, i.e. test-strips which, like in a pregnancy test, detect the presence of a disease or biomarker, are promising devices for fast, cost-effective and reliable healthcare. Compared to polymers, paper holds the additional promise of being easy and environmentally friendly in terms of disposal.
Paper-based analytical devices such as lateral flow immunoassays (LFIA) usually consist of a sample-receiving zone into which the test-liquid is applied, a labeling zone where a labelled biomolecule specifically and strongly binds to the detectable biomarker from the test-liquid, and a capture zone where the labelled biomarker/antibody complex is bound and forms a visually detectable pattern (e.g. a stripe on the paper). A fourth zone, the control zone, ensures that the assay strip is fully functional even when the result of the detection zone is negative. Specific zones and connecting channel structures can be made by treating the paper with wax, so that no test liquids will enter the impregnated parts. Barriers also enable running the assays in a parallelised manner on one strip. However, classical wax barriers are time-consuming since they are printed in a line-by-line fashion, much like printing toner on a conventional printer. They are also breakable in that when the paper is folded, the barrier cracks and leaks.
In response to such issues, we have developed a reagent mixture that can be photostructured and renders the paper hydrophobic at the desired spots (Fig. 2E). This technology allows generating paper-based devices via photostructuring methods, thereby creating complex flow assays which constrain the liquid flow to given sections of the paper. The resulting barriers are soft and can be bent without damaging the overall assay.
Reliable surface functionalisation
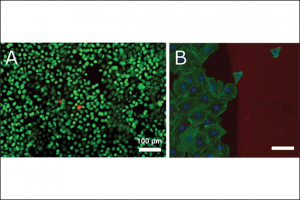
The biofunctionalisation of surfaces is a key aspect in healthcare research. Here, technical substrates (like microtiter plates or chips) are in direct contact with the microscopic biological world (like biomolecules, cells and micro-organisms). Interaction, manipulation and communication between these worlds are only possible with the right surface modifications. Cells will grow on certain areas depending on the surface chemistry, while they will largely avoid areas with different chemistry (Fig. 4B). Some cell types only grow on special surface geometries with a microenvironment closely matching the extracellular matrix of the living organism. Cleverly positioned molecules on the surface can even induce the directed movement of cells on the surface. Decorating surfaces with the adequate molecules and functions is intricate. Due to energy imbalances, surfaces always behave differently from the corresponding bulk materials.
For a cell to sense a pattern of biomolecules the patterns must be in the range of the cell size with feature sizes well below its own length scale. Most human cell types are significantly smaller than 100 µm. Micromolecular patterns below that size can be achieved most conveniently by light structuring. We have built a maskless lithography system that uses a micromirror array with about a million individually addressable mirrors that can be used to project structured light onto a surface. By radical reactions, the projected light induces the binding of labelled biomolecules to the substrate surface, if an adequate binding layer is present. This mechanism can be used to bind any type of biomolecule (DNA; proteins and enzymes, antibodies) and small molecules (biotin) to the surface.
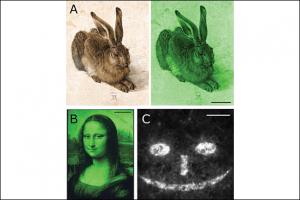
We have created various patterns on all the materials reported herein: PS, PMMA, glass and paper (Fig. 3), and we have shown that the production of the aforementioned paper-based LFIA strips can be significantly facilitated by protein patterning. Individual zones of a paper-based LFIA are conventionally made from individual materials, e.g. a highly hydrophilic material for the sample receiving zone, or nitrocellulose for the zones containing the biomolecules of
the strip. The zones have to be joined by pressure and the highly brittle nitrocellulose has to be protected by polymer casings. Nitrocellulose is largely used due to the fact that biomolecules adsorb non-specifically to its hydrophobic surface. With our technique we can immobilise antibodies directly onto the paper, abolishing the need for different materials and enabling the fabrication of test strips in paper as the sole material.
Conclusion
Providing material platforms that combine a broad range of materials with processing and structuring methods, as well as reliable surface (bio-) functionalisation, is a major factor in healthcare research, diagnostics and clinical testing. Controlling surface structures and interface chemistry is the key to communicating with cells and organisms. Our group has developed various tools to address these questions on a wide range of length scales, materials and applications.
NeptunLab’s work has been partly funded by the German Federal Ministry of Education and Research (BMBF), funding code: 03X5527 and 031A095C
Further reading
-
- F Kotz et al. Three-dimensional Printing of Transparent Fused Silica Glass, Nature, 544, 337-339, 2017
- F Kotz et al. Liquid Glass: A Facile Soft Replication Method for Structuring Glass, Advanced Materials, 2016
- TM Nargang et al. Functionalisation of paper using photobleaching: a fast and convenient method for creating paper-based assays with colorimetric and fluorescent readout, Engineering in Life Sciences, 2016
- TM Nargang et al. Liquid polystyrene: a room-temperature photocurable soft lithography compatible pour-and-cure-type polystyrene, Lab Chip (special issue Emerging Investigators), 14, 2698-2708, 2014
- D Helmer et al. Suspended Liquid Subtractive Lithography: One-step generation of 3D channel geometries in viscous curable polymer matrices, Nature Scientific Reports, 2017, DOI: 10.1038/s41598-017-07630-w
- Waldbaur et al. Maskless projection lithography for the fast and flexible generation of greyscale protein patterns, Small, 8, 10, 1570-1578, 2012