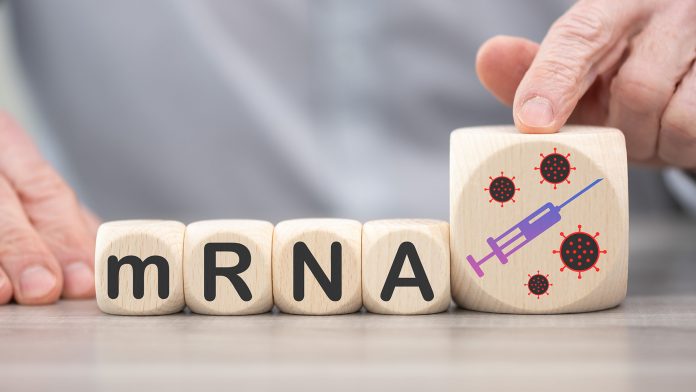
Following the unprecedented development of vaccines during the COVID-19 pandemic, we spoke to Dinah Knotts about mRNA vaccine technology and what further opportunities it can offer in fighting infectious diseases.
Dinah Knotts has been working in the clinical research industry for over 25 years and is now Vice President of Project Management at ICON where she leads the company’s Vaccines and Infectious Diseases group. As part of her role, she led the operational delivery of the first Pfizer COVID vaccine study which led to an Emergency Use Authorization in an extraordinary 247 days and full FDA approval. Lorna Rothery spoke to her about mRNA vaccine technology, how it was readily adapted to aid the COVID-19 pandemic response and what opportunities there are for its use in treating other infectious diseases.
Can you provide a brief overview of how mRNA vaccine technology was first developed and what led to its use in the coronavirus vaccines?
In 1990, scientists discovered that mouse cells would temporarily produce specific proteins if the mouse was injected with corresponding mRNA – the substance that directs protein production in cells. This discovery planted the seed for mRNA vaccines. If animal cells could be instructed to synthesise viral or bacterial proteins, it would provoke an immune response, priming the immune system to recognise and destroy any pathogens presenting that protein in the future. This would offer a novel method of vaccination, where the immune system could be trained to combat a specific pathogen without needing to survive an active infection.
The idea of mRNA vaccines garnered a lot of initial excitement because the method offered an elegant and more versatile alternative to conventional vaccines, which use inactive pathogen or pathogen components to directly stimulate an immune response. However, despite this excitement, there were many hurdles to overcome before mRNA vaccines could be implemented in humans. The primary challenge was that the body recognised injected mRNA as foreign and, therefore, degraded it immediately, before it could instruct protein synthesis.
Decades of advancements were necessary to develop a more stable mRNA molecule and a protective delivery vehicle. A lipid nanoparticle that would protect the injected mRNA and shuttle it into cells was first developed in 2000 and first tested as a delivery mechanism for an mRNA vaccine in 2012. Researchers also found that chemically modifying mRNA, by replacing uridine nucleotides with pseudouridine, helped prevent an immune response to injected mRNA.
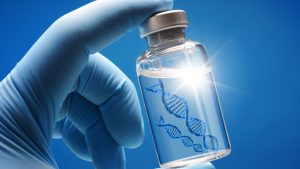
When the COVID-19 pandemic began, researchers pursued vaccine development using every available platform. By this point, mRNA vaccination technology was well-established, and production was quicker and more easily scalable than alternative vaccination methods. In addition, much of the work needed to identify and sequence the target protein for COVID-19 was already completed during the preclinical development of vaccines against other coronaviruses – SARS-CoV and MERS-CoV. Thanks to converging research efforts and substantial funding, it took less than a year from when SARS-CoV-2 was isolated in January 2020 to develop the mRNA vaccine.
How does the immune response to mRNA vaccines and traditional vaccines differ?
To be effective and safe, a vaccine must be able to provoke a strong, targeted immune response, without posing a real threat to the recipient. To accomplish this, conventional vaccines contain a dead or weakened pathogen, or pathogen components, which directly provokes an immune response. In cases where the immune response is expected to be weak (such as with an inactivated vaccine, where the pathogen is destroyed), the vaccine may include compounds to help make the immune response stronger.
Unlike conventional vaccines, mRNA vaccines provoke the immune system indirectly by instructing healthy cells to temporarily produce a foreign protein, which then triggers a targeted immune response. This foreign protein resembles the protein of a target pathogen. However, the vaccine, itself, does not include any part of that pathogen. For example, the COVID-19 mRNA vaccine informs cells to produce a protein that resembles the SARS-CoV-2 ‘spike protein’. The subsequent immune response primes the immune system to recognise and attack SARS-CoV-2 more efficiently in the future.
How do mRNA vaccines differ from traditional vaccines? What advantages does the technology offer?
The manufacturing process for conventional vaccines requires the collection, growth and shipment of a pathogen or its components. In the case of viral diseases, viruses – or parts of viruses – need to be grown in chicken eggs or mammalian cells. This manufacturing process can take months and requires specialised labs.
Meanwhile, mRNA vaccines only require that the pathogen be isolated, and its target protein be sequenced during initial development. The pathogen is not needed following development, because manufacturers do not have to produce or purify the protein directly. The mRNA is synthesised, cell-free, from a DNA template. Manufacturing an mRNA vaccine takes only a week and uses standard laboratory materials.
The production process for mRNA vaccines is especially advantageous for the development of vaccines against emerging infectious diseases. Manufacturing can be scaled and standardised across vaccine types because only the mRNA sequence encoding the target protein needs to be replaced to make a new vaccine. The versatility of mRNA vaccines also makes it possible to develop vaccines against non-infectious, immune-mediated diseases, such as autoimmune disorders and cancers.
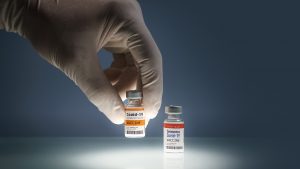
What scope is there for the development of mRNA vaccines to treat other infectious diseases?
Along with the ability to combat emerging infectious diseases, the rapid development and deployment of mRNA vaccine technology make them well-suited for targeting pathogens that mutate quickly. The primary reason that the duration of immune protection varies between pathogens is the rate that pathogens replicate. A pathogen with a slow rate of replication will not change as much over time. So, immune protection from a vaccine is likely to be long-lasting. Conversely, if a pathogen changes rapidly or has many variants, the immune system may struggle to recognise and rapidly respond to that pathogen, even after repeated exposure. For example, influenza has 20 subtypes that vary in prevalence over time, while measles has one strain that has remained mostly unchanged. Accordingly, a vaccine against measles often provides lifelong immunity, while vaccines against influenza are recommended yearly.
Flu vaccines would be able to provide longer-lasting immunity if they could introduce the immune system to multiple subtypes of the virus at the same time. As mentioned, there are 20 known subtypes of influenza, and it is difficult to predict which subtype will be most prevalent in a future flu season. Due to the complexities and costs of manufacturing conventional vaccines, it has not been feasible to make a single flu vaccine against all 20 subtypes. However, mRNA vaccines can theoretically incorporate instructions for multiple proteins at the same time. The recent success of mRNA vaccines against COVID-19 has bolstered the efforts to develop a universal influenza vaccine with mRNA technology that would immunise against all major subtypes.
Successful mRNA vaccines for COVID-19 have also paved the way for the treatment of numerous infectious diseases, such as HIV, tuberculosis and Zika, and the technology may also prove useful for the treatment of autoimmune diseases and cancers. While the field of mRNA vaccine research is in its infancy, the technology’s versatility, scalability, and speed of development make it especially promising.
Why do some vaccines deliver long-lasting immune protection and others do not?
It is important to note that there are additional reasons why the immune protection of vaccines differs, beyond a pathogen’s mutation rate. For example, depending on the initial strength of the immune response to a vaccine, immune protection may be longer lasting after multiple encounters with the target protein or pathogen, rather than just one. In this case, boosters are used to increase a vaccine’s effectiveness. A vaccine’s ability to protect against disease also varies because every person’s immune system is different. A very young, old, or weakened immune system may not be effective at preventing infection, even if it mounts a targeted response against a pathogen. However, when vaccines do not prevent infection, they are able to make it less severe or deadly than it would have been otherwise.
This article is from issue 25 of Health Europa Quarterly. Click here to get your free subscription today.