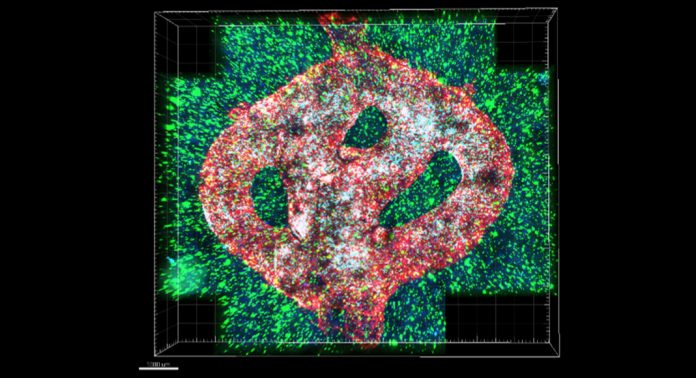
Scientists have used 3D printing biotechnology to create a complex model of a glioblastoma tumour.
Researchers from Tel Aviv University in Israel have used 3D bioprinting to create a complex system of blood vessel-like tubes through which blood cells and drugs can flow, mirroring a real tumour. The team hope that this model will lead to breakthroughs in cancer research.
The findings from this research have been published in the journal Science Advances.
The study was led by Professor Ronit Satchi-Fainaro, Director of the Morris Kahn 3D-BioPrinting for Cancer Research Initiative at Tel Aviv University, and the technology was created by PhD student Lena Neufeld, alongside other researchers at Professor Satchi-Fainaro’s laboratory. The 3D-bioprinted models are based on samples from patients, taken directly from operating rooms at the Tel Aviv Sourasky Medical Center.
The study was designed with the aim of enabling a more complex and thorough research method, to go beyond the typical studies of cells on 2D surfaces.
Professor Satchi-Fainaro said: “Glioblastoma is the most lethal cancer of the central nervous system, accounting for most brain malignancies.
“In a previous study, we identified a protein called P-Selectin, produced when glioblastoma cancer cells encounter microglia – cells of the brain’s immune system. We found that this protein is responsible for a failure in the microglia, causing them to support rather than attack the deadly cancer cells, helping the cancer spread. However, we identified the protein in tumours removed during surgery, but not in glioblastoma cells grown on 2D plastic petri dishes in our lab. The reason is that cancer, like all tissues, behaves very differently on a plastic surface than it does in the human body. Approximately 90% of all experimental drugs fail at the clinical stage, because the success achieved in the lab is not reproduced in patients.”
With this problem in mind, the researchers created the first 3D-bioprinted model of a glioblastoma tumour, which includes 3D cancer tissue surrounded by extracellular matrix, which communicates with its microenvironment via functional blood vessels.
“It’s not only the cancer cells. It’s also the cells of the microenvironment in the brain; the astrocytes, microglia, and blood vessels connected to a microfluidic system – namely a system enabling us to deliver substances like blood cells and drugs to the tumour replica.
“Each model is printed in a bioreactor we have designed in the lab, using a hydrogel sampled and reproduced from the extracellular matrix taken from the patient, thereby simulating the tissue itself. The physical and mechanical properties of the brain are different from those of other organs, like the skin, breast, or bone. Breast tissue consists mostly of fat, bone tissue is mostly calcium; each tissue has its own properties, which affect the behaviour of cancer cells and how they respond to medications. Growing all types of cancer on identical plastic surfaces is not an optimal simulation of the clinical setting,” added Professor Satchi-Fainaro.
Predicting effective treatment for individuals
After successfully printing the 3D tumour, Professor Satchi-Fainaro and her colleagues demonstrated that the 3D-bioprinted model had the potential to achieve much more than cells grown on petri dishes. The researchers concluded that the 3D-bioprinted model may be able to predict the most suitable treatment for a specific patient.
“We proved that our 3D model is better suited for prediction of treatment efficacy, target discovery, and drug development in three different ways. First, we tested a substance that inhibited the protein we had recently discovered, P-Selectin, in glioblastoma cell cultures grown on 2D petri dishes, and found no difference in cell division and migration between the treated cells and the control cells which received no treatment. In contrast, in both animal models and in the 3D-bioprinted models, we were able to delay the growth and invasion of glioblastoma by blocking the P-Selectin protein. This experiment showed us why potentially effective drugs rarely reach the clinic simply because they fail tests in 2D models, and vice versa: why drugs considered a phenomenal success in the lab, ultimately fail in clinical trials.
“In addition, collaborating with the lab of Dr Asaf Madi of the Department of Pathology at TAU’s Faculty of Medicine, we conducted genetic sequencing of the cancer cells grown in the 3D-bioprinted model, and compared them to both cancer cells grown on 2D plastic and cancer cells taken from patients. Thus, we demonstrated a much greater resemblance between the 3D-bioprinted tumours and patient-derived glioblastoma cells grown together with brain stromal cells in their natural environment. Through time, the cancer cells grown on plastic changed considerably, finally losing any resemblance to the cancer cells in the patient’s brain tumour sample. The third proof was obtained by measuring the tumour growth rate. Glioblastoma is an aggressive disease partially because it is unpredictable: when the heterogeneous cancer cells are injected separately into model animals, the cancer will remain dormant in some, while in others, an active tumour will develop rapidly. This makes sense because we, as humans, can die peacefully of old age without ever knowing we have harboured such dormant tumours. On the dish in the lab, however, all tumours grow at the same rate and spread in the same rate. In our 3D-bioprinted tumour, the heterogeneity is maintained and development is similar to the broad spectrum that we see in patients or animal models.”
Personalised medicine
Professor Satchi-Fainaro also said that the approach could also enable the development of new drugs, as well as discovery of new drug targets – at a much faster rate than today. The team also hope that the technology will facilitate personalised medicine for patients.
“If we take a sample from a patient’s tissue, together with its extracellular matrix, we can 3D-bioprint from this sample 100 tiny tumours and test many different drugs in various combinations to discover the optimal treatment for this specific tumour. Alternately, we can test numerous compounds on a 3D-bioprinted tumour and decide which is most promising for further development and investment as a potential drug. But perhaps the most exciting aspect is finding novel druggable target proteins and genes in cancer cells – a very difficult task when the tumour is inside the brain of a human patient or model animal. Our innovation gives us unprecedented access, with no time limits, to 3D tumours mimicking better the clinical scenario, enabling optimal investigation,” Professor Satchi-Fainaro added.